Introduction
In this piece we’re going to cover a recent article by Wackerhage et al. (2018) titled, “Stimuli and sensors that initiate skeletal muscle hypertrophy following resistance exercise.” This paper is the most current review of how and why a muscle grows in response to resistance training. With this article, our goal is to break it down and disseminate some of the information to make it more digestible for the lay person. If you’re interested in the full paper, you can read it here.
The review itself is separated into individual stimuli that may sensitize certain sensors and/or receptors that then signal a cascade of effects that leads to growth. Here, we’re just going to focus on the stimuli themselves as individual sensors and signals within the body are incredibly complex and far outside the boundary of, “need to know,” information for the majority of people. That means our main goal is to describe the current science on the mechanisms of inducing hypertrophy. This is an important discussion as it’s imperative to have a solid understanding of the different ways you can cause growth and how to best use that knowledge in the weightroom. Let’s get started.
Stimulus 1: Mechanical Load/Tension
Mechanical tension is probably the most important determinant of muscle hypertrophy (34). This is most easily seen when comparing the training of a bodybuilder to a marathon runner. A marathon runner, in all technicalities, undergoes significantly greater training volumes and experiences similar levels of muscles damage compared to bodybuilders. So why is the bodybuilder more muscular? Lifting weights! Resistance training induces large amounts of mechanical load/tension on the muscle which is a primary stimulus for hypertrophy (34).
Why is mechanical tension such a strong driver of hypertrophy? Think of the environment we live in; what’s a constant force/stressor we have to deal with? Gravity. Over time, our bodies have adapted and evolved to better handle the constant force of gravity. That’s why we have such well-developed musculoskeletal systems – dealing with the constant mechanical load of gravity triggered this development. Therefore, our bodies are very good at responding to mechanical loads. We have several sensors that are responsible for detecting loads and forces and these sensors can generate growth signals as a response to constant increased loads like a training program would produce (34).
We see the importance of mechanical loading in immobilized patients – when an individual is bedridden, significant muscle atrophy (loss of size) occurs as the muscle is never utilized nor has to exert large amounts of force (27). We also see this in other studies where scientists were able to mechanically load muscle fibers without inducing muscle damage or metabolite accumulation – the muscle fibers still grew even without other potential hypertrophy stimuli (15). A common way we see this is in the forearm muscles of individuals who perform continuous work with their hands, such as carpenters or mechanics – Popeye has nothing on those people.
So how can you use this knowledge in your own training? There’s two main ways to induce large amounts of mechanical tension and subsequent growth. The first is to lift heavier weights. Studies show that subjects training in the 60-90% 1RM range had much a higher protein synthesis response to training than subjects who used lighter loads (18). The second way is to lift lighter weights to failure – studies show that lifting lighter weights to failure is just as effective at inducing hypertrophy as lifting heavier weights (20). Use both strategies in your training to create optimal amounts of tension on the muscle and force it to respond with growth. Check out our Time Under Tension article here for a more in-depth discussion on why both of these strategies can help boost growth.
Stimulus 2: Metabolic Stress
Metabolic stress refers to the accumulation of metabolites in the muscle during fatiguing exercise. These metabolites, namely lactate and hydrogen ions, accumulate because the muscle is working more than relaxing which makes it nearly impossible to clear out the metabolites (34).
The first sign that metabolic stress may be a stimulus for growth was seen in immobilized hospital patients. We know that immobilization can cause muscle loss (27), but researchers found that if you occluded an immobilized limb, much like using blood flow restriction in training, the limb did not lose as much muscle mass as limbs that were not occluded (32). Other researchers took this a step further and found that blood flow restriction, in combination with resistance training, helped induce even more growth than just training alone (1,21).
So why does metabolic stress help gains so much? There’s a few theories to this method. The first, and most difficult to prove, is that metabolite accumulation in and of itself acts as a sensor that drives growth signals (34). Another, and much more plausible theory, is that we see an increase in muscle activation when metabolites begin to accumulate in the muscle – probably due to both a reduction in cellular pH and acceleration of motor unit fatigue (8). This increase in muscle activation can induce mechanical tension on more muscle fibers which would cause more growth when compared to similar exercise with less muscle activation (34). The last theory is that the accumulation of metabolites causes cell swelling, much like an intense muscle pump. This cell swelling exerts a type of mechanical tension on the muscle fiber from the inside-out that may signal to the cell a need to expand and grow to better withstand this stress (29).
So how can you increase metabolic stress in training? Besides blood flow restriction training (31), you can train at lighter loads with higher reps and shorter rest periods (34). This causes the muscle to perform a ton of work with very little relaxation time which leads to metabolite accumulation. Sleds are a great tool for inducing high amounts of metabolic stress with needing blood flow restriction. Another interesting way to use this method is by using blood flow restriction on your heaviest training days. Since metabolite accumulation can increase muscle activation (8), you may get a better overall training stimulus from lifting heavy loads when compared to heavy lifting without BFR (19,22,24). One caveat is that you’ll probably want to keep sets to a minimum and only use BFR on one or two exercises during a heavy day. Too much metabolite accumulation can increase muscle fatigue which will impair your abilities to lift heavy. Several top powerlifters, such as Matt Wenning and Chris Duffin, have used this method to great success in their training.
Stimulus 3: Muscle Damage
The last potential stimulus for hypertrophy is muscle damage. Muscle damage was probably one of the first theories as to why a muscle grows (34) and that’s why many people have heard the classic, “break a muscle down and build it up stronger,” bit. Over the last decade or so we’ve seen a ton of mounting evidence against muscle damage as a stimulus for hypertrophy, so let’s get into the details on that.
Muscle damage is a result of performing new exercises or heavy eccentric loading through a full range of motion (26,28). This damage is microscopic in nature, mostly affecting the structural components of a muscle fiber (5). When a muscle fiber is damaged, we see an inflammation response by the body, increased protein breakdown, and even increased muscle enzymes, like creatine kinase, leak into the bloodstream from the damaged cell (17). Experienced lifters typically undergo less muscle damage than novel lifters as they’re not performing new exercises as often and have built adaptation to eccentric training (34).
The main issue with examining the effects muscle damage may have on hypertrophy is that you can’t really isolate muscle damage as a stimulus with a resistance training protocol (34). Mechanical tension is the main driver of hypertrophy and muscle damage is typically a byproduct of a tension overload – so it’s tough to say the effect damage could have on hypertrophy in lieu of tension. We do see some potential evidence for damage leading to growth when we examining training at certain muscle lengths/ranges of motion. We know that training at a greater muscle length (larger range of motion) creates more muscle damage (3,26). Training at greater muscle lengths also leads to more muscle growth than restricted range of motion training (4,25). Some have interpreted this as a link between the two, but it’s probably more so due to the fact that full range of motion training will induce more mechanical tension on more muscle fibers due to the length-tension property of skeletal muscle (11).
Another scenario in which some have claimed a link is the fact that eccentric overload training causes more muscle damage and leads to slightly greater muscle growth than concentric training (9,30). An easy argument to this theory, however, is that eccentric overload training causes greater mechanical tension to the muscle which, again, would dispel muscle damage as a precursor to growth (10,23).
The last theory that damage may lead to growth is the fact that muscle damage can increase satellite cell recruitment (6). Satellite cell recruitment is, for the most part, necessary for muscle hypertrophy when considering the Nuclear Domain Theory. This theory states that a cell nucleus can only control a finite amount of cell space. If a cell undergoes growth, the cell will need to recruit more nuclei to maintain control of the cell space. Satellite cells can donate their nuclei to muscle fibers to allow for growth to occur (34) – we cover this theory much more in depth in our Muscle Memory article here if you’re interested in more info. A stick in the wheel of this thought is that non-damaging exercise can also lead to satellite cell recruitment (7), so damage itself might not be so special after all.
Lastly, let’s look at the mounting evidence against muscle damage as a determinant to hypertrophy. One of the first ways we started seeing this was in studies showing that muscle damage did not correlate to increases in muscle protein synthesis (14). An increase in muscle protein synthesis is typically an immediate marker used to show that muscle hypertrophy is occurring, so a lack of protein synthesis response to exercise would allow one to suffice that the muscle is not growing. Other studies found that study groups undergoing damaging exercise did not experience more muscle growth than groups who did not experience muscle damage due to exercise (12). The last hiccup in the damage theory is again, a comparison to long distance running. Long distance running causes vast amounts of muscle damage but does not result in muscle growth (16). Some studies even show muscle cells shrinking in response to a long distance running program (33). I don’t think any bodybuilder wants to look like a marathon runner, so maybe muscle damage shouldn’t be the primary focus of training.
Like we already mentioned, it’s tough to isolate muscle damage as a determinant of growth since mechanical tension is the primary cause of muscle damage. However, it is possible to do so when considering muscle injury. Theoretically, you could stab yourself in the thigh and expect growth if muscle damage alone was, indeed, a stimulus for hypertrophy. That’s clearly not advisable and injuries have been shown to not cause growth following repair in rodent studies (34).
Lastly, lifters will typically experience soreness as a sign of muscle damage but the severity of muscle soreness is not well-correlated with the amount of muscle damage one experiences (34). The takeaway here is that your soreness after a training session is not indicative of the quality of the workout. If you’re not sore following a workout, that doesn’t mean you’re not growing! In the same thought pattern, extreme soreness following training does not mean you had a great session and may even impair gains as you won’t be able to train with the same frequency due to intense soreness (13). You don’t have to actively try to avoid muscle damage by any means – it’s going to occur when you’re training hard and using full ranges of motion. Just understand that damage probably isn’t a primary driver of hypertrophy. Focus on increasing mechanical tension through heavy weights or training to failure, and use metabolic stress methods when you’re feeling run down from lifting heavy/to failure all of the time.
Conclusion
This is a summary of our current scientific knowledge as to what makes a muscle grow. The takeaway here is to really focus a good portion of your training on inducing as much mechanical tension to the muscle as possible. Check out the Time Under Tension article here for multiple ideas on how to achieve this. When you need a break from all the heavy weights or training near failure, training strategies that promote metabolic stress are a great idea for the bodybuilder wanting to continue making gains during a deload period. And lastly, using your post-workout muscle soreness as an indicator for a previous workout’s effectiveness isn’t the best method. Muscle damage doesn’t appear to be necessary for growth and the degree of your soreness doesn’t always correlate with how much damage you experience. Just keep in mind that muscle damage is not bad in any way and will most likely occur following training methods that optimize mechanical tension – which is important for gains!
References
- Abe, T., Loenneke, J. P., Fahs, C. A., Rossow, L. M., Thiebaud, R. S., & Bemben, M. G. (2012). Exercise intensity and muscle hypertrophy in blood flow–restricted limbs and non‐restricted muscles: a brief review. Clinical Physiology and Functional Imaging, 32(4), 247-252.
- (2009). American College of Sports Medicine position stand. Progression models in resistance training for healthy adults. Medicine & Science in Sports & Exercise, (41), 687-708.
- Baroni, B. M., Pompermayer, M. G., Cini, A., Peruzzolo, A. S., Radaelli, R., Brusco, C. M., & Pinto, R. S. (2017). Full range of motion induces greater muscle damage than partial range of motion in elbow flexion exercise with free weights. The Journal of Strength & Conditioning Research, 31(8), 2223-2230.
- Bloomquist, K., Langberg, H., Karlsen, S., Madsgaard, S., Boesen, M., & Raastad, T. (2013). Effect of range of motion in heavy load squatting on muscle and tendon adaptations. European Journal of Applied Physiology, 113(8), 2133-2142.
- Clarkson, P. M., & Hubal, M. J. (2002). Exercise-induced muscle damage in humans. American Journal of Physical Medicine & Rehabilitation, 81(11), S52-S69.
- Crameri, R. M., Aagaard, P., Qvortrup, K., Langberg, H., Olesen, J., & Kjær, M. (2007). Myofibre damage in human skeletal muscle: effects of electrical stimulation versus voluntary contraction. The Journal of Physiology, 583(1), 365-380.
- Crameri, R. M., et. al. (2004). Changes in satellite cells in human skeletal muscle after a single bout of high intensity exercise. The Journal of Physiology, 558(1), 333-340.
- Dankel, S. J., Mattocks, K. T., Jessee, M. B., Buckner, S. L., Mouser, J. G., & Loenneke, J. P. (2017). Do metabolites that are produced during resistance exercise enhance muscle hypertrophy? European Journal of Applied Physiology, 117(11), 2125-2135.
- Douglas, J., Pearson, S., Ross, A., & McGuigan, M. (2017). Chronic adaptations to eccentric training: a systematic review. Sports Medicine, 47(5), 917-941.
- Eliasson, J., Elfegoun, T., Nilsson, J., Kohnke, R., Ekblom, B., & Blomstrand, E. (2006). Maximal lengthening contractions increase p70 S6 kinase phosphorylation in human skeletal muscle in the absence of nutritional supply. American Journal of Physiology-Endocrinology and Metabolism, 291(6), E1197-E1205.
- Finni, T., Ikegawa, S., Lepola, V., & Komi, P. V. (2003). Comparison of force–velocity relationships of vastus lateralis muscle in isokinetic and in stretch‐shortening cycle exercises. Acta Physiologica Scandinavica, 177(4), 483-491.
- Flann, K. L., LaStayo, P. C., McClain, D. A., Hazel, M., & Lindstedt, S. L. (2011). Muscle damage and muscle remodeling: no pain, no gain? Journal of Experimental Biology, 214(4), 674-679.
- Foley, J. M., Jayaraman, R. C., Prior, B. M., Pivarnik, J. M., & Meyer, R. A. (1999). MR measurements of muscle damage and adaptation after eccentric exercise. Journal of Applied Physiology, 87(6), 2311-2318.
- Gibala, M. J. (2000). Nutritional supplementation and resistance exercise: what is the evidence for enhanced skeletal muscle hypertrophy? Canadian Journal of Applied Physiology, 25(6), 524-535.
- Goldberg, A. L., Etlinger, J. D., Goldspink, D. F., & Jablecki, C. (1975). Mechanism of work-induced hypertrophy of skeletal muscle. Medicine and Science in Sports, 7(3), 185-198.
- Hikida, R. S., Staron, R. S., Hagerman, F. C., Sherman, W. M., & Costill, D. L. (1983). Muscle fiber necrosis associated with human marathon runners. Journal of the Neurological Sciences, 59(2), 185-203.
- Komulainen, J., & Vihko, V. (1994). Exercise-induced necrotic muscle damage and enzyme release in the four days following prolonged submaximal running in rats. Pflügers Archiv, 428(3-4), 346-351.
- Kumar, V., et. al. (2009). Age‐related differences in the dose–response relationship of muscle protein synthesis to resistance exercise in young and old men. The Journal of Physiology, 587(1), 211-217.
- Kurobe, K., Huang, Z., Nishiwaki, M., Yamamoto, M., Kanehisa, H., & Ogita, F. (2015). Effects of resistance training under hypoxic conditions on muscle hypertrophy and strength. Clinical Physiology and Functional Imaging, 35(3), 197-202.
- Lasevicius, T., et. al. (2018). Effects of different intensities of resistance training with equated volume load on muscle strength and hypertrophy. European Journal of Sport Science, 1-9.
- Loenneke, J. P., Wilson, J. M., Marín, P. J., Zourdos, M. C., & Bemben, M. G. (2012). Low intensity blood flow restriction training: a meta-analysis. European Journal of Applied Physiology, 112(5), 1849-1859.
- Manimmanakorn, A., Hamlin, M. J., Ross, J. J., Taylor, R., & Manimmanakorn, N. (2013). Effects of low-load resistance training combined with blood flow restriction or hypoxia on muscle function and performance in netball athletes. Journal of Science and Medicine in Sport, 16(4), 337-342.
- Moore, D. R., Phillips, S. M., Babraj, J. A., Smith, K., & Rennie, M. J. (2005). Myofibrillar and collagen protein synthesis in human skeletal muscle in young men after maximal shortening and lengthening contractions. American Journal of Physiology-Endocrinology and Metabolism, 288(6), E1153-E1159.
- Nishimura, A., Sugita, M., Kato, K., Fukuda, A., Sudo, A., & Uchida, A. (2010). Hypoxia increases muscle hypertrophy induced by resistance training. International Journal of Sports Physiology and Performance, 5(4), 497-508.
- Noorkõiv, M., Nosaka, K., & Blazevich, A. J. (2015). Effects of isometric quadriceps strength training at different muscle lengths on dynamic torque production. Journal of Sports Sciences, 33(18), 1952-1961.
- Paulsen, G., Ramer Mikkelsen, U., Raastad, T., & Peake, J. M. (2012). Leucocytes, cytokines and satellite cells: what role do they play in muscle damage and regeneration following eccentric exercise? Exercise Immunology Review, 18.
- Psatha, M., et. al. (2012). A longitudinal MRI study of muscle atrophy during lower leg immobilization following ankle fracture. Journal of Magnetic Resonance Imaging, 35(3), 686-695.
- Schoenfeld, B. J. (2012). Does exercise-induced muscle damage play a role in skeletal muscle hypertrophy? The Journal of Strength & Conditioning Research, 26(5), 1441-1453.
- Schoenfeld, B. J. (2013). Potential mechanisms for a role of metabolic stress in hypertrophic adaptations to resistance training. Sports Medicine, 43(3), 179-194.
- Schoenfeld, B. J., Ogborn, D. I., Vigotsky, A. D., Franchi, M. V., & Krieger, J. W. (2017). Hypertrophic effects of concentric vs. eccentric muscle actions: a systematic review and meta-analysis. The Journal of Strength & Conditioning Research, 31(9), 2599-2608.
- Suga, T., et. al. (2009). Intramuscular metabolism during low-intensity resistance exercise with blood flow restriction. Journal of Applied Physiology, 106(4), 1119-1124.
- Takarada, Y., Takazawa, H., & Ishii, N. (2000). Applications of vascular occlusions diminish disuse atrophy of knee extensor muscles. Medicine and Science in Sports and Exercise, 32(12), 2035-2039.
- Trappe, S., Harber, M., Creer, A., Gallagher, P., Slivka, D., Minchev, K., & Whitsett, D. (2006). Single muscle fiber adaptations with marathon training. Journal of Applied Physiology, 101(3), 721-727.
- Wackerhage, H., Schoenfeld, B. J., Hamilton, D. L., Lehti, M., & Hulmi, J. J. (2018). Stimuli and sensors that initiate skeletal muscle hypertrophy following resistance exercise. Journal of Applied Physiology.
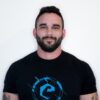
From being a mediocre athlete, to professional powerlifter and strength coach, and now to researcher and writer, Charlie combines education and experience in the effort to help Bridge the Gap Between Science and Application. Charlie performs double duty by being the Content Manager for The Muscle PhD as well as the Director of Human Performance at the Applied Science and Performance Institute in Tampa, FL. To appease the nerds, Charlie is a PhD candidate in Human Performance with a master’s degree in Kinesiology and a bachelor’s degree in Exercise Science. For more alphabet soup, Charlie is also a Certified Strength and Conditioning Specialist (CSCS), an ACSM-certified Exercise Physiologist (ACSM-EP), and a USA Weightlifting-certified performance coach (USAW).