Introduction
We all know that one guy in the gym – the one who boasts about how he used to bench press 500-lbs and ran a 4.3-second 40yd dash. Ball State University Director of Strength and Conditioning, Jason Roberson, sums this silliness up nicely: “Have you ever even stood in the same room as someone who can bench press 500-lbs or run a sub-4.4 40? They don’t have to tell you that they can do that. You just know by being near them.”
We hear people throw around numbers like this all of the time casually, but the reality is that having the ability to achieve these things is essentially unheard of. In the grand scheme of things, almost no one benches over 500-lbs. And even fewer people can run a sub-4.4 40yd dash. While intense training for years and years can play a major role in both strength and athletic accomplishments, a genetic component absolutely exists. Not everyone has the inherent ability to throw around massive amounts of weight or to sprint amongst the cheetahs in the plains of Africa. Genetics play a massive role in determining many of the following, but instead of focusing on individual genes and confusing biology terms, we’re going to look at the bigger picture of why elite athletes are so good.
Genetic Determinants of Strength
Most of the main determinants of strength are biomechanically-based. We’ll cover a few topics here, but many of the topics in the Genetic Determinants of Athletic Performance also relate to this section. The primary concept to understand here is joint torque – this is how fast and/or powerfully a joint can move based off the force that a muscle applies to it. The force that a muscle applies to a joint is based off of a few things, but a major player is the attachment site of the muscle in accordance to the joint that it’s moving (13).
Muscle attachment sites are probably one of the primary differences between someone who can bench press 500-lbs and someone who cannot. Studies have shown that attachment site locations can vary widely between subjects (6) which can directly impact the torque produced by a certain joint. An easy example to see how muscle attachment sites may impact joint torque is by examining the biceps action at the elbow joint (review here). You can also review this in our Physics of Bodybuilding article (here).
The biceps are a class 3 lever system, in which both the muscle attachment and resistance are on the same side of the elbow joint. Many muscles in the human body work as class 3 lever systems so the biceps are an easy example to apply this logic to (9). The main point to understand here is that the weight in your hand also produces torque on the elbow in the opposite direction of your biceps muscle. Now, torque is equal to the distance the force is applied from the joint multiplied by the force that is applied – AKA the weight in your hand multiplied by the distance from the weight to your elbow. The distance from the joint to the force is a huge determinant of torque, and in the class 3 lever it’s easy to see that the biceps attach rather close to the joint. Therefore, the biceps have to produce a ton of force to overcome the torque caused by the weight in your hand since the muscle attaches so close to the joint.
Now, imagine if you could move that biceps attachment just a little further down the forearm. Now any force that you produce with your biceps muscle can induce that much more torque on the elbow joint since the attachment is further away. See that guy at the gym that can curl 185lbs+ for reps no problem? His biceps might attach further down his forearm than most other people which gives him a massive mechanical advantage to lift large weights.
An interesting way to infer this logic is through orthopedic research examining how to reconstruct joints and/or soft tissue attachments. Studies have actually shown that changing the attachment site of a tendon to a less mechanically-advantageous position can reduce the range of motion of a joint and joint torque at various positions (24).
So what determines muscle attachment sites? Genetics (19). If you’re weak and have a hard time getting stronger, blame your parents. Powerlifters and Olympic weightlifters obviously work their asses off in training, but the best of the best have a major genetic advantage. World record holders are born, it’s just up to them to train enough to realize their true potential.
Strength can also be somewhat determined by muscle fiber type (18). We know that type II/fast twitch muscle fibers produce more force and power than slow twitch fibers – primarily because they are larger in size. Therefore, individuals with slightly higher proportions of fast twitch fibers may have a genetic advantage when it comes to lifting weights and being stronger. Fiber type composition does appear to play a larger role in sprinting performance than strength sport performance, however (4).
Genetic Determinants of Muscle Hypertrophy
The genetics of building muscle is incredibly complex and involves all kinds of hormones and sensors/signalers that are, frankly, outside the, “need to know,” scope of this piece. We’ll stick to two main genetic components here that are of major interest to bodybuilders – myostatin and satellite cells.
Myostatin is a gene that may influence that amount of muscle an individual has and can grow. Genetic mutations can cause reductions in myostatin signaling or other myostatin disruptions that can result in much larger than normal levels of muscle hypertrophy (17). Myostatin deficiencies are common in some types of bulls such as the Belgian Blue – click the link here and scroll down to the photo to see what a myostatin deficiency can lead to.
Some human cases of myostatin deficiencies have been shown (17). It has also been proposed by many a fitness layperson that professional bodybuilders more than likely have some sort of myostatin mutation that allows them to build and maintain such muscle mass. If you look at the, “before,” pictures of many current IFBB pros, you’ll see that the majority of them were pretty jacked as teenagers, too.
The concept of myostatin deficiency directly affects another genetic aspect that more than likely plays a large role in one’s ability to build muscle: satellite cell recruitment. Satellite cells are essentially the stem cells of muscle – when muscle fibers experience large amounts of tension or damage, satellite cells are activated to help sort out the mess and provide assistance in the adaptation process. The procedure of activating satellite cells is probably due to the Nuclear Domain Theory, of which we cover in greater detail in the Muscle Memory article (read here). Essentially, for a muscle fiber to grow, more nuclei will be required to control the new cell space (1). Satellite cells can donate their nuclei to muscle cells to allow the growth adaptation to take place (16).
Now, the problem here is that research has shown that satellite cell recruitment can vary widely between subjects (15,21). Scientists have posed the theory that satellite cell activation is a genetic factor (3) so some people simply recruit satellite cells better than others and therefore have a greater growth response to exercise (16). We all have that one friend or know that one individual who can put on muscle like nobody’s business. Odds are their bodies naturally recruit satellite cells at a much higher rate than the rest of us. It’s okay to be jealous; I am too.
Genetic Determinants of Athleticism
Gifted athletes will probably possess all of the traits mentioned in this article – that’s why the best of the best get paid insane amounts of money to do what they do. Besides strength and muscle building factors, elite athletes probably have a few other unique differences as well.
Many people assume that extremely fast athletes are ridiculously fast twitch fiber oriented, and that certainly seems to ring true. Elite sprinters do contain more fast twitch fibers than sedentary individuals with the typical difference being around 20% (4,20). In more recent work, elite Olympic weightlifters have also been shown to posses high levels of fast twitch muscle fibers (here). Sprint and power-based sports definitely appear to be dominated by fast twitch athletes, but let’s read more into this.
One of the main proposals as to why an elite sprinter is so fast is not necessarily how quickly their muscles can contract, but instead, how quickly their muscles can relax during contractions. As a muscle fiber contracts, proteins slide past one another by creating cross bridges that, “pull,” along one another. These cross bridges can only pull one short stroke before having to dissociate and rebind at another site to continue the muscle contraction. The process of dissociating and rebinding is termed, “muscle relaxation,” and the faster that one can relax their muscle fibers, the faster the muscle will shorten and more overall power will be produced (22). This process is mediated by multiple enzymes within the muscle that are necessary for ATP resynthesis, calcium binding, and all sorts of complicated processes (22).
Famed Soviet sport performance coach, Yuri Verkhoshansky, believed that naturally talented sprinters respond to training mainly by improving relaxation rates as opposed to actual muscle strength (22). Data from other studies supports Verkhoshansky’s assertion by finding that running velocity is highly correlated with anaerobic enzyme activity in the muscle that would directly affect ATP resynthesis (11). Unfortunately for the rest of us slow pokes, relaxation rates appear to be highly hereditary as studies have shown that neither age nor gender have any correlation with muscle relaxation rates (12). So next time someone comments that Usain Bolt is faster than you, you can respond by saying, “No, he just relaxes faster than me.” See how that one works out for you.
Another determinant of athletic performance may be tendon hysteresis. Tendon hysteresis refers to the efficiency with which a tendon absorbs and redirects force (8). Tendons are the connective tissue between muscle and bone, they stretch when a muscle stretches and contract when a muscle shortens. Therefore, a tendon’s ability to efficiently transmit force from lengthening into shortening can determine how much overall power can be transferred to the bone and overall locomotion (8).
On the other hand, some have proposed that tendon stiffness can be a determinant of athletic power. Tendon stiffness refers to the ability of a tendon to stretch and reflex to produce/transmit force, but not the efficiency with which it does so. Studies have found that tendon stiffness may be a predictor of athletic performance, but tendon hysteresis has been shown to have a much wider range of biological values (7). Therefore, there may not be a significant difference between the stiffness of a normal individual’s tendons and a freak athlete’s tendons, but there could definitely be an extreme difference in the hysteresis of the tendons between the two. There’s a reason why even the shortest of NBA players can dunk a basketball and you can barely jump over a deck of cards. At least you can still blame your parents.
Genetic Determinants of Endurance Performance
Directly related to the idea of tendon hysteresis is the running economy of elite endurance athletes. It has been theorized throughout the years that elite marathon runners are simply better at dissipating heat than other runners (5,14). Two factors can play into this, the first being tendon hysteresis.
Since hysteresis is a measurement of efficiency, what happens to the energy that an inefficient tendon loses? It gets lost as heat (8). An inefficient runner, then, may experience more heat accumulation due, in part, to poor tendon hysteresis which accelerates the fatigue process during long distance running.
Efficient runners will also get more bang-for-their-buck from their metabolism. Several enzymes within the muscle are necessary for determining metabolic rates during exercise and the greatest of endurance athletes may simply have more active enzymes (14). We see this in studies that find that higher performing endurance runners can maintain higher running velocities at lower VO2 levels (23) – this means that they literally get more work done out of less fuel. Kind of like using a small turbocharged engine over a larger naturally aspirated engine – more power and better gas mileage. Great runners are turbocharged, just without the cool badges and spoilers.
Some of the running efficiency may be due to a preponderance of slow twitch muscle fibers in elite endurance athletes (20). Slow twitch muscle fibers are more aerobic in nature and would not accumulate metabolic byproducts to the same extent as fast twitch muscle fibers. Therefore, slow twitch fibers will fatigue more slowly and will also produce less heat than fast twitch fibers.
One last, but very important, limiting factor of endurance performance is oxygen delivery to the working muscle (2). This can obviously be improved by cardiovascular training, but some people are still naturally inclined to deliver more oxygen to their muscles. This is why men typically have a greater VO2 max than women – men usually have higher hematocrit levels which allows the blood to carry more oxygen (2). This explains the blood doping epidemic that we saw in cycling during the reign of Lance Armstrong. Blood doping allows cyclists to increase the hematocrit in their blood to improve oxygen carrying capacity and delivery to working muscles.
Another determinant of oxygen delivery that may have more genetic basis is the capillary density surrounding a muscle (2,10). Capillaries are the site of oxygen exchange between the vascular system and the muscle. This is where oxygen is delivered to the muscle and metabolic waste products are removed (10). Thus, it’s easy to recognize that the more capillaries an individual has in a square inch of muscle tissue, the more oxygen can be delivered and the more metabolic waste can be flushed. Endurance training can increase capillary density, but this can also be highly determined by genetics (10).
Conclusion
At the end of the day, there’s several factors that make elite athletes who they are, this is just a peek at some of the more noticeable ones. These specific traits can also determine what sport an individual excels at; ideal muscle attachments and relaxation rates can lead to incredible sprinting abilities while running economy and capillary density can help produce high-performing marathon runners. Unfortunately, not many people are blessed with any of these genetic factors, let alone multiple of them. You can spend time being jealous of the people who were born with these abilities, or you can continue to make yourself the best version of you that you possibly can. Training adaptations can absolutely improve some of the factors we talked about above, so you can still continue to progress your own performance and get one step closer to the genetic elite. Just understand that there are definitely some underlying differences that you might never be able to make up for. The only solution is to build a time machine and pick new parents, and frankly, that sounds like more trouble than it’s worth.
References
- Allen, D. L., Roy, R. R., & Edgerton, V. R. (1999). Myonuclear domains in muscle adaptation and disease. Muscle & Nerve, 22(10), 1350-1360.
- Bassett, D. R., & Howley, E. T. (2000). Limiting factors for maximum oxygen uptake and determinants of endurance performance. Medicine and Science in Sports and Exercise, 32(1), 70-84.
- Bouchard, C., & Rankinen, T. (2001). Individual differences in response to regular physical activity. Medicine and Science in Sports and Exercise, 33(6 Suppl), S446-51.
- Costill, D. L., Daniels, J., Evans, W., Fink, W., Krahenbuhl, G., & Saltin, B. (1976). Skeletal muscle enzymes and fiber composition in male and female track athletes. Journal of Applied Physiology, 40(2), 149-154.
- Coyle, E. F. (2007). Physiological regulation of marathon performance. Sports Medicine, 37(4-5), 306-311.
- Duda, G. N., Brand, D., Freitag, S., Lierse, W., & Schneider, E. (1996). Variability of femoral muscle attachments. Journal of Biomechanics, 29(9), 1185-1190.
- Farris, D. J., Trewartha, G., & McGuigan, M. P. (2011). Could intra-tendinous hyperthermia during running explain chronic injury of the human Achilles tendon? Journal of Biomechanics, 44(5), 822-826.
- Finni, T., Peltonen, J., Stenroth, L., & Cronin, N. J. (2013). On the hysteresis in the human Achilles tendon. American Journal of Physiology-Heart and Circulatory Physiology, (114), 515-517.
- Hamilton, N. P. (2011). Kinesiology: scientific basis of human motion. Brown & Benchmark. Dubuque, IA.
- Joyner, M. J., & Coyle, E. F. (2008). Endurance exercise performance: the physiology of champions. The Journal of Physiology, 586(1), 35-44.
- Komi, P. V., Rusko, H., Vos, J., & Vihko, V. (1977). Anaerobic performance capacity in athletes. Acta Physiologica Scandinavica, 100(1), 107-114.
- Lennmarken, C., Bergman, T., Larsson, J., & Larsson, L. E. (1985). Skeletal muscle function in man: force, relaxation rate, endurance and contraction time-dependence on sex and age. Clinical Physiology (Oxford, England), 5(3), 243-255.
- Lieber, R. L., & Shoemaker, S. D. (1992). Muscle, joint, and tendon contributions to the torque profile of frog hip joint. American Journal of Physiology-Regulatory, Integrative and Comparative Physiology, 263(3), R586-R590.
- Marino, F. E., Lambert, M. I., & Noakes, T. D. (2004). Superior performance of African runners in warm humid but not in cool environmental conditions. Journal of Applied Physiology, 96(1), 124-130.
- Petrella, J. K., Kim, J. S., Cross, J. M., Kosek, D. J., & Bamman, M. M. (2006). Efficacy of myonuclear addition may explain differential myofiber growth among resistance-trained young and older men and women. American Journal of Physiology-Endocrinology and Metabolism, 291(5), E937-E946.
- Petrella, J. K., Kim, J. S., Mayhew, D. L., Cross, J. M., & Bamman, M. M. (2008). Potent myofiber hypertrophy during resistance training in humans is associated with satellite cell-mediated myonuclear addition: a cluster analysis. Journal of Applied Physiology, 104(6), 1736-1742.
- Schuelke, M., Wagner, K. R., Stolz, L. E., Hübner, C., Riebel, T., Kömen, W., … & Lee, S. J. (2004). Myostatin mutation associated with gross muscle hypertrophy in a child. New England Journal of Medicine, 350(26), 2682-2688.
- Tesch, P. A., Wright, J. E., Vogel, J. A., Daniels, W. L., Sharp, D. S., & Sjödin, B. (1985). The influence of muscle metabolic characteristics on physical performance. European Journal of Applied Physiology and Occupational Physiology, 54(3), 237-243.
- Thomis, M. A. I., Beunen, G. P., Leemputte, M. V., Maes, H. H., Blimkie, C. J., Claessens, A. L., … & Vlietinck, R. F. (1998). Inheritance of static and dynamic arm strength and some of its determinants. Acta Physiologica Scandinavica, 163(1), 59-71.
- Thorstensson, A., Larsson, L., Tesch, P., & Karlsson, J. (1977). Muscle strength and fiber composition in athletes and sedentary men. Medicine and Science in Sports, 9(1), 26-30.
- Timmons, J. A. (2010). Variability in training-induced skeletal muscle adaptation. Journal of Applied Physiology, 110(3), 846-853.
- Verkhoshansky, Y. V. (1996). Quickness and velocity in sports movements. New Studies in Athletics, 11, 29-38.
- Weston, A. R., Mbambo, Z., & Myburgh, K. H. (2000). Running economy of African and Caucasian distance runners. Medicine and Science in Sports and Exercise, 32(6), 1130-1134.
- Yamamoto, N., Itoi, E., Tuoheti, Y., Seki, N., Abe, H., Minagawa, H., … & Okada, K. (2007). Glenohumeral joint motion after medial shift of the attachment site of the supraspinatus tendon: a cadaveric study. Journal of Shoulder and Elbow Surgery, 16(3), 373-378.
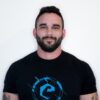
From being a mediocre athlete, to professional powerlifter and strength coach, and now to researcher and writer, Charlie combines education and experience in the effort to help Bridge the Gap Between Science and Application. Charlie performs double duty by being the Content Manager for The Muscle PhD as well as the Director of Human Performance at the Applied Science and Performance Institute in Tampa, FL. To appease the nerds, Charlie is a PhD candidate in Human Performance with a master’s degree in Kinesiology and a bachelor’s degree in Exercise Science. For more alphabet soup, Charlie is also a Certified Strength and Conditioning Specialist (CSCS), an ACSM-certified Exercise Physiologist (ACSM-EP), and a USA Weightlifting-certified performance coach (USAW).