Introduction
The subject of discussion in this article won’t have a ton of application in the gym. It’s more-or-less a, “hey, this is a thing that happens,” piece rather than a, “here’s some science and how to apply it,” like we usually do. Regardless, this is an incredibly interesting topic that, someday, might develop some useful application in the gym. We still don’t have enough information on the topic to develop a ton of takeaways for the layperson but, nevertheless, knowledge is power and we’re all here to become stronger.
So, now that 75% of readers have already clicked out of this article, the remaining 25% of us get to cover architectural gear ratios in skeletal muscle. Sounds fun, right? Let’s dig in.
Background
Before we get into the muscle part of things, let’s first get some background information on gear ratios. One of the most common applications we often hear gear ratios being discussed is when talking about cars.
In a car, the gear ratio is simply the ratio of the size of the input gear (pinion, driveshaft, etc.) and the output gear (differential, transaxle, etc.). For example, a 2:1 gear ratio would mean that, for every 2 turns of the input gear, we get 1 turn of the output gear. For a driveshaft and transaxle example, the driveshaft turns the transaxle at a given gear ratio that ultimately rotates the wheels of the car to speed up the vehicle.
Now, most people understand that cars have multiple gears in their transmission (electric cars and CVTs are outside this discussion…). These gears differ in size and, therefore, interact with the driveshaft with different ratios. Lower gears, like first and second, typically have higher gear ratios as they are designed for acceleration; however, higher gears will have lower gear ratios so that it requires less work by the engine to maintain a given speed.
Ultimately, several factors determine the proper gear ratios in a given vehicle and, frankly, those are well outside the scope of this piece and my small automotive mechanics realm of knowledge. However, now we get to move to the slightly more relevant topic at hand: muscle gear ratios.
Fast and Furious… Muscles?
Before covering muscle gear ratios, we need to cover some of the more common muscle architecture types in the human body. As far as the major muscle groups are concerned, the most common architecture types are parallel and pennate. Parallel muscles exhibit muscle fibers that run parallel to one another and insert directly into their tendon. The biceps brachii are a great example of these muscles. On the other hand, we have pennate muscles that attach to their tendon “obliquely,” or at an angle (2). Muscles can be uni-, bi-, or multipennate depending on how many different angles they attach at. The quadriceps, gastrocnemius, and deltoids are all examples of pennate muscles.
A key benefit to pennate architecture is that, for a given area of muscle, you can pack more muscle fibers in a pennate muscle as opposed to a parallel muscle. Think of this like a parking lot with angled spaces – the entire purpose is to fit more spaces into the parking lot! Now, when you look at the picture to the left, you can see that, as a pennate muscle contracts, the angle of its muscle fibers in accordance to the tendon also changes. This is due to the muscle changing shape but needing to maintain constant volume (3). What does this mean?
The shortening (contraction) of the muscle fibers changes the angle with which they insert onto the tendon. This means that, as the muscle fibers shorten, they also slightly rotate around their attachment to the tendon. Therefore, since muscle fibers are both shortening and rotating, the contraction speed of the whole muscle is different than the contraction speed of single muscle fibers (2,4,9,10,14).
With this in mind, pennate muscles exhibit a unique “gearing” in which their gear ratio is the contractile speed of the entire muscle compared to the contractile speed of a single muscle fiber (5). In a normal muscle, this ratio would simply be 1:1; however, since pennate fibers “rotate” while they shorten, the contractile speed of the muscle as a whole can exceed the contractile speed of individual muscle fibers which results in a gear ratio higher than 1:1 (5).
Training Applications?
Well, not really. Not yet, anyways. We do have studies showing that, as a muscle grows in response to training, its pennation angle typically increases (1,7,13). On the flip side, when muscle size reduces following significant detraining, pennation angles typically decrease (11). What is the advantage to increasing pennation angle?
Just like the parking lot concept we touched on earlier, increasing pennation angle allows more muscle fibers to be packed into a given area (1). However, this benefit only occurs up until about 45° because any angle beyond that will likely reduce the number of muscle fibers in a given area (1).
So, realistically, there’s not much application here other than acknowledging some unique things that are going on in your body that you likely didn’t previously know about. It’s worth keeping in mind, though, that increasing pennation angle increases the amount of muscle fibers in a given area which can help boost overall strength gains (1,7). This is one reason why the quads are stronger than the hamstrings; obviously, the quads are just larger in general and have more a more favorable attachment (relative to the knee), but the pennation angles in the individual quadriceps muscles also allow the quads to pack more muscle fibers into a given area.
One last unique aspect of pennate muscles is that they typically have shorter muscle fibers and, therefore, don’t contract as quickly as parallel fibers (5,8). Therefore, these slower contraction speeds can help pennate muscles experience more tension in a given movement (2) and might mean that they are easier to grow than parallel muscles. When examining the quads and hamstrings, it’s quite possible to make that distinction as many have overdeveloped quads while their hamstrings lag behind. Several factors can play into this but the pennation of the quads could certainly contribute!
Conclusion
So, this really doesn’t represent much of a, “how to,” scenario in which we explain how you can increase pennation angles and influence muscle gear ratios to make some kind of gains. However, it’s an interesting scenario that shows that muscle adaptations to exercise can take many forms. The architectural changes, such as pennation angle, are something we don’t touch on as much because they’re not as applicable as other types of gains. But, like we said before, knowledge is power, and understanding developing sciences can help broaden your exercise science knowledge in general.
References
- Aagaard, P., Andersen, J. L., Dyhre‐Poulsen, P., Leffers, A. M., Wagner, A., Magnusson, S. P., … & Simonsen, E. B. (2001). A mechanism for increased contractile strength of human pennate muscle in response to strength training: changes in muscle architecture. The Journal of Physiology, 534(2), 613-623.
- Azizi, E., Brainerd, E. L., & Roberts, T. J. (2008). Variable gearing in pennate muscles. Proceedings of the National Academy of Sciences, 105(5), 1745-1750.
- Baskin, R. J., & Paolini, P. J. (1967). Volume change and pressure development in muscle during contraction. American Journal of Physiology-Legacy Content, 213(4), 1025-1030.
- Brainerd, E. L., & Azizi, E. (2005). Muscle fiber angle, segment bulging and architectural gear ratio in segmented musculature. Journal of Experimental Biology, 208(17), 3249-3261.
- Eng, C. M., Azizi, E., & Roberts, T. J. (2018). Structural determinants of muscle gearing during dynamic contractions. Integrative and Comparative Biology, 58(2), 207-218.
- Fouré, A., Nordez, A., & Cornu, C. (2012). Effects of eccentric training on mechanical properties of the plantar flexor muscle-tendon complex. Journal of Applied Physiology, 114(5), 523-537.
- Kawakami, Y., Abe, T., & Fukunaga, T. (1993). Muscle-fiber pennation angles are greater in hypertrophied than in normal muscles. Journal of Applied Physiology, 74(6), 2740-2744.
- Lieber, R. L., & Fridén, J. (2000). Functional and clinical significance of skeletal muscle architecture. Muscle & Nerve: Official Journal of the American Association of Electrodiagnostic Medicine, 23(11), 1647-1666.
- Maganaris, C. N., Baltzopoulos, V., & Sargeant, A. J. (1998). In vivo measurements of the triceps surae complex architecture in man: implications for muscle function. The Journal of Physiology, 512(2), 603-614.
- Muhl, Z. F. (1982). Active length‐tension relation and the effect of muscle pinnation on fiber lengthening. Journal of Morphology, 173(3), 285-292.
- Narici, M., Franchi, M., & Maganaris, C. (2016). Muscle structural assembly and functional consequences. Journal of Experimental Biology, 219(2), 276-284.
- Potier, T. G., Alexander, C. M., & Seynnes, O. R. (2009). Effects of eccentric strength training on biceps femoris muscle architecture and knee joint range of movement. European Journal of Applied Physiology, 105(6), 939-944.
- Timmins, R. G., Shield, A. J., Williams, M. D., Lorenzen, C., & Opar, D. A. (2016). Architectural adaptations of muscle to training and injury: a narrative review outlining the contributions by fascicle length, pennation angle and muscle thickness. British Journal of Sports Medicine, 50(23), 1467-1472.
- Zuurbier, C. J., & Huijing, P. A. (1992). Influence of muscle geometry on shortening speed of fibre, aponeurosis and muscle. Journal of Biomechanics, 25(9), 1017-1026.
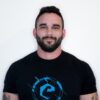
From being a mediocre athlete, to professional powerlifter and strength coach, and now to researcher and writer, Charlie combines education and experience in the effort to help Bridge the Gap Between Science and Application. Charlie performs double duty by being the Content Manager for The Muscle PhD as well as the Director of Human Performance at the Applied Science and Performance Institute in Tampa, FL. To appease the nerds, Charlie is a PhD candidate in Human Performance with a master’s degree in Kinesiology and a bachelor’s degree in Exercise Science. For more alphabet soup, Charlie is also a Certified Strength and Conditioning Specialist (CSCS), an ACSM-certified Exercise Physiologist (ACSM-EP), and a USA Weightlifting-certified performance coach (USAW).