Introduction
We often hear that using a full range of motion (ROM) in a given exercise is necessary for growth. With this in mind, quarter squatters get laughed out of the squat rack and the skinny bros cheating their way through endless sets of curls never make any gains. However, is this actually the case? Do you need to train each muscle through a full range of motion to make gains?
While it seems trivial to both beginners and veterans alike, this is an incredibly important question that, when answered, could really help optimize training and reduce wasted reps. Before we can really get into the nitty gritty, though, we need to go over the primary mechanism at play here: the length-tension curve.
The Length-Tension Curve
We know that mechanical tension is probably the most important stimulus for muscle growth (Wackerhage et al., 2019). Muscles detect mechanical tension through mechanoreceptors that can sense things like forces and changes in the muscle fiber’s shape (Burkholder, 2007). There are several things that determine how much tension a muscle can experience in an exercise, with range of motion being one of them. Range of motion affects muscle tension through an interaction known as the, “Length-Tension Curve.”
The length-tension curve describes how much active (and passive) tension a muscle can produce in respect to its length (Lieber et al., 2017). The curve is most often designed according to sarcomere length. Sarcomeres are the individual contractile units of a muscle fiber; when sarcomeres shorten, the muscle shortens. As the length-tension curve shows on the right, muscles should produce their peak force around their resting length as each sarcomere will have an optimal amount of actin and myosin overlap. Actin and myosin are the primary contractile proteins in a sarcomere and slide past one another to shorten or lengthen the muscle fiber. Too few overlaps (bridges) and you can’t produce enough force – AKA, the muscle is too long. When the muscle is too short, you have structural interferences within the sarcomere that block crossbridge formation (Lieber et al., 2017).
Now, as a muscle lengthens, passive components also play a role to increase overall tension well beyond the plateau portion of the curve. This includes elastic structures like connective tissue and fascia as well as the giant protein, titin, which all work together to add additional tension at long muscle lengths – this leads to the longest muscle lengths having the most total tension a muscle can experience. As the giant protein, titin, stretches in longer muscle lengths, it can also mediate the tension signals produced by mechanoreceptors (Kruger & Kotter, 2016) which may induce a larger growth stimulus. With all of these benefits in mind, many (including us) have advocated training muscles through a full ROM to ensure that a muscle experiences its highest level of tension.
Is this actually necessary?
What does this Mean for Training?
If you have an exercise science degree, or are currently studying for one, you’re probably [hopefully] familiar with the concept of the length-tension curve. However, despite what many were told in school, this curve does not perfectly represent all muscles. In fact, just about every muscle group occupies a different portion on the length-tension curve, and not every muscle is actually active throughout the entire curve like we were all told in school. If you check the samples on the left, you can see that these two example muscles are actually active on different portions of the curve.
Why is this important for training? Well, since each muscle is different, this means that we might have to use different ranges of motion on certain muscles to promote growth. Training with a full ROM is actually more important for some muscles, whereas other muscles it might not matter as much. In fact, we have studies showing that full ROM squats are better for quad growth than partial ROM squats (Bloomquist et al., 2013; Kubo et al., 2019). However, we also have studies showing that full ROM curls are no more effective at developing the biceps than partial ROM curls that didn’t even achieve a full stretch (Pinto et al., 2012).
Why is this the case? Well, as you’ll see in the next section, some muscles are active well into the ascending portion of the curve. This means that the muscle can get shorten too much and experience active insufficiency (Buckthorpe et al., 2019). Active insufficiency essentially means that a muscle is active, but is too short to produce significant force or experience significant tension. A classic example that many know of is the gastrocnemius during seated calf raises. Since the gastroc also crosses the knee joint, performing calf raises with the knee bent shortens the gastroc too much, leading to active insufficiency (Hébert-Losier et al., 2012).
With these discrepancies in mind, it’s worth exploring the length-tension curve specific to all of the major muscle groups to better identify how to train each one. Let’s dig in.
Biceps
We might as well start with the most important muscle group, right? As you can see in the curve on the right, the biceps are mostly active on the plateau and ascending portions of the curve (Koo et al., 2002; Murray et al., 2000). Why is this the case? Well, the “resting position” of the biceps is pretty much a fully-elongated position; the biceps can’t really stretch beyond that unless you hyperextend your elbow (not recommended). What does this mean for training?
The biceps are one of the few muscle groups where we have some range of motion training studies. One study from Pinto et al. (2012) found that 10-weeks of full ROM (0-130° flexion) biceps training led to similar growth compared to 10-weeks of partial ROM (50-100° flexion) training. Since the biceps aren’t active on the descending portion of the curve, it’s unlikely that they have to be trained at long lengths for growth. But, if that’s the case, why do all the bros doing cheat curls still not make gains?
It’s important to also understand that the biceps are active on the ascending portion of the curve. This means that the biceps can experience active insufficiency, especially in positions where we have shoulder and elbow flexion occurring at the same time (Schoenfeld, 2002). What do we see when the bros are cranking out their awful curls? Tons of shoulder flexion. AKA, no gains! Keep in mind, though, that preacher curls involve passive shoulder flexion in which your biceps don’t have to be actively flexing the shoulder. Therefore, preacher curls are still great for biceps development.
The takeaway here is that, even know the biceps might not have to be trained at longer lengths, we still recommend using a strict, full range of motion on most biceps exercises to ensure you don’t get too much shoulder flexion involved during your biceps training. Knowing these little tricks is incredibly important due to the mass amounts of misinformation available online, like this Instagram post here advising people to flex their shoulders at the top of a curl. This will do nothing for biceps growth.
Biceps Update!
Great news! Another ROM study just came out assessing elbow ROM and biceps gains. We’ll spare you the nitty gritty details, but essentially, Sato et al. (2021) had subjects perform two different ranges of motion while performing preacher curls; one group did curls at the bottom half of the range of motion while the other group used the top half. Intriguingly, this study actually found that performing curls at the bottom half of the ROM was more effective for growth than the top half! Does this debunk using the length-tension curve in regard to biceps training? Not quite. You see, one main difference between this study and the above study from Pinto et al. (2012) was the ROM used in the partial/top half condition. Pinto et al. (2012) used 50-100 degrees of elbow flexion, but Sato et al. (2021) used 80-130 degrees. Since the biceps are active on the ascending portion of the length-tension curve, it’s actually plausible that isolating the absolute top of the range of motion does result in active insufficiency, thus impairing biceps gains. Keep in mind for your own cheat curls!
Triceps
Next up, we have the triceps. We often hail the biceps as the kings of the upper arm, but the triceps actually make up a greater percentage of upper arm muscle mass than the biceps, so it’s important to develop your horseshoes correctly for maximum, “sun’s out, guns out” capabilities.
As you can see in the curve on the left, the triceps are mostly active on the plateau portion of the curve (Murray et al., 2000). This means that the triceps should be trained through a full range of motion, but it also means that the triceps probably don’t experience active insufficiency like the biceps. Now, we only have one head of the triceps (long head) that actually crosses two joints. However, you can see in the curve that the long head barely travels down the ascending curve. This means that things like dumbbell kickbacks can still develop the long head of the triceps even though it’ll be shortened at both ends in the exercise. This is useful as this shoulder position is typically where the long head can contribute the most to elbow extension force (Landin et al., 2018).
The triceps are another muscle group that we have a few range of motion training studies to discuss. Interestingly enough, both studies found that any range of motion can be used for triceps growth! Goto et al. (2019) found that partial triceps extensions (45-90°) produced more growth than full ROM triceps extensions (0-120°) over an 8-week training program. In addition, Stasinaki et al. (2018) found that a group doing stretched triceps extensions (70-150°) experienced similar growth to a group performing shortened triceps extensions (10-90°) over a 6-week training program.
With this in mind, we recommend using multiple ranges of motion when training triceps, and we also recommend using ranges of motion that keep tension on the triceps throughout the movement. This can be done by foregoing full lockout on things like skullcrushers or rope pushdowns. Keep tension on those horseshoes and force them to grow!
Lats
Next up, we have the lats. This one shouldn’t come as a surprise to many as the curve on the right shows that the lats are mostly active on the plateau and descending portions of the curve (Gerling & Brown, 2013). Combined with the moment arms of the lats (Hik & Ackland, 2019), this leads to the lats being much stronger at longer lengths than they are at shorter lengths – this is why just about anyone can do the first half of a pull-up, but getting your chin over the bar is where it gets tricky.
With this in mind, we recommend training the lats at longer lengths as much as possible. Get a big stretch on things like pulldowns, pull-ups, and even straight arm pulldowns. When it comes to rowing movements, make sure you’re really “reaching” for the floor to exaggerate the stretch. We also like landmine row variations or even T-bar row variations as these exercises are typically “heavier” at the bottom due to the angle of the bar or machine. This helps create a greater challenge for the lats at longer lengths where they’re stronger. This is also helpful for those last few reps in a set to failure – just because you didn’t do a full rep doesn’t mean you didn’t get a good lat training stimulus. Just make sure you get a full stretch on each rep!
Pectorals
Ah yes, time to get to the ol’ pectorals. Instead of the classic curve, we actually have an odd-looking bar chart for the pecs, courtesy of Garner and Pandy (2003). Since this isn’t the full curve, we added some of our own notes to help these bars make a little more sense. In short, besides the pec minor, all portions of the pectorals are active on every part of the length-tension curve. This means that using all ranges of motion is necessary for pec training, but it’s especially important in the stretched position where we can take advantage of both passive and active tension. Multiple other studies have found similar results (Wolfe et al., 1992; Wu et al., 2016) in a variety of populations. However, those with tighter pecs (i.e. not as active on descending portion) might be more susceptible to injury (Wolfe et al., 1992). Therefore, always listen to your body while training!
It’s important to note, though, that the pecs are also active on the ascending portion of the curve (especially the middle chest), which means that they might experience some active insufficiency at short lengths. There’s not many exercises that involve this, but one such is something we call a Pinch Press (some might call it a lying Svend Press here). This exercise involves “pinching” two dumbbells together while performing a dumbbell bench range of motion. This promotes a nasty chest pump as you really shorten the fibers of the chest, but you’ll notice that you can’t do nearly as much weight as you could on a normal dumbbell bench. Why? Because the pecs have become actively insufficient. If you like this exercise a lot, we suggest using it on an incline bench to really focus on the upper chest as you’ll get more shoulder flexion in this position.
All-in-all, use a full ROM on chest exercises and make sure you’re getting plenty of a stretch on every rep – especially for isolation movements like dumbbell or cable flyes.
Shoulders
We get our shoulder information from that same Garner and Pandy study from 2003, hence the bars, rather than the normal curve. As you can see, all portions of the delts are active on the plateau and ascending portions, but only the anterior and lateral delts are active on the descending portion the curve.
Therefore, it’s probably prudent to use a mostly lengthened or neutral range of motion on most delt exercises – you’ll definitely want to avoid training the delts at shorter lengths. With this in mind, exercises like front or lateral raises should only be done to the point where your arm is parallel to the floor; any higher and you’re likely achieving active insufficiency in the muscle. Conversely, the posterior delt is likely to get pretty sore from using larger ranges of motion since it is not active on the descending portion of the curve (Brockett et al., 2002).
All-in-all, this is probably why we see many bodybuilders have success with training the shoulders in somewhat limited ranges of motion, like not achieving lockout on overhead presses or only using a limited ROM in front or lateral raises. If you’re an athlete, it’s a different story since you’re training motions, rather than muscles. But if muscular development is all that you seek, you probably don’t need a full ROM with the delts, at least at the top of the movement, anyways.
Traps
Again, we only have the Garner and Pandy (2003) study with the fancy bars to understand where the traps are on the length-tension curve. As we can see, all portions of the traps are mostly active on the ascending and plateau portions of the curve. However, we often recommend that people squeeze their traps at the top of a shrug, so what gives?
It’s important to keep in mind that the middle and upper traps elevate and upward rotate the scapula during shrugging motions. Therefore, the traps actually don’t shorten to a massive degree in a shrug. The traps are probably at their shortest in exercises like overhead press where we have full elevation and upward rotation. I don’t think anyone does an overhead press for trap development, so I don’t think this is a muscle group that we really have to worry about active insufficiency with, even though it could technically occur.
The only exercise we might be a little concerned about with active insufficiency and the traps is something like an upright row. You might achieve enough elevation and upward rotation to place the traps in active insufficiency. Since the lateral delts also achieve active insufficiency at short lengths, we recommend getting a good stretch on upright rows, but there’s no need to raise your elbows higher than parallel to the floor.
When it comes to horizontal rowing exercises, we’d definitely recommend getting a good stretch and focusing on retracting your shoulder blades to hit the traps during rows. However, as your shoulder blades pinch together, your traps will likely experience less tension due to active insufficiency. With the traps and lats in mind, most rowing movements probably don’t *need* to be performed to full shoulder extension to get the best training stimulus for the muscles involved.
Quads
As shown in the curve, ¾ of the quad muscles are mostly active on the plateau and descending portion of the curve (Son et al., 2018) which would suggest that using a larger ROM during leg exercises is better for quad growth. Indeed, we have multiple studies showing that deep squats induce more quad growth than partial squats (Bloomquist et al., 2013; Kubo et al., 2019).
However, that curve only covers ¾ of the quad muscles, so what about the rectus femoris? Research shows that rectus femoris is active on all portions of the curve (Cutts, 1988) which makes perfect sense as it is a biarticulate muscle. Rectus femoris achieves active insufficiency in movements where hip flexion and knee extension happen simultaneously (Murdock & Agyeman, 2020), like a straight leg raise or something similar. Therefore, if you want to isolate rectus femoris, we suggest only flexing the hip or extending the knee, but don’t combine both. The only situation in which that’s fine is a seated leg extension in which the hip is passively flexed. Since rectus femoris doesn’t have to be actively flexing the hip, it can still produce sufficient knee extension force (Watanabe et al., 2012).
Hamstrings
Next up, we have the hamstrings. Before we get into their odd-looking graphs (the best we could find, unfortunately), it’s worth reviewing some quick hamstrings anatomy. The hamstrings consist of 3 different muscles, the biceps femoris (2 muscle heads), the semitendinosus, and semimembranosus. As you can see in the three graphs shown, each muscle has a slightly different length-tension relationship. Both the semimembranosus and biceps femoris (A & B) are active on all portions which means that these muscles should be trained at longer lengths, but they also will likely experience active insufficiency (Kellis et al., 2012; Wan et al., 2017).
The semitendinosus is the only hamstrings muscle that likely doesn’t experience much active insufficiency, which means it might also play a role in exercises like glute bridges or hip thrusts where hip extension is occurring with a flexed knee. On the flip side, though, full hamstrings development will require things like leg curls, deadlift variations, and good mornings. Since all hamstrings muscles are active on the descending portion of the curve, we recommend using longer muscle lengths to train the hamstrings. Make sure you get a good stretch on things like leg curls or glute ham raises and really exaggerate the stretch on exercises like stiff leg deadlifts or good mornings.
Hamstrings Update
Exciting news! A new study was published recently that explored hamstrings growth in subjects performing seated and lying leg curls. As expected, the seated leg curl resulted in greater hypertrophy than the lying leg curl (Maeo et al., 2020). Why is this the case? The hamstrings are active on the descending portion of the length-tension curve, meaning they’re probably better trained at long lengths! Since a lying leg curl will shorten the proximal end of the hamstrings (due to extended hips), the hamstrings won’t be trained at as long of a length.
Luckily for us, Maeo et al., (2020) also published much better-looking length-tension curves than the oddball graphs above. We’ll include those here but these simply offer further evidence for the hamstrings being mostly active on the plateau and descending portions of the curve.
Glutes
We searched for days but, unfortunately, couldn’t find any information on the glutes. This is a tricky muscle group to research as there isn’t much on the glutes outside physical therapy journals or Dr. Bret Contreras’ work. A good portion of data on other muscles in this article is actually derived from surgical journals where information that can be beneficial to joint reconstruction is incredibly important. Despite this catastrophic disappointment, we can use some of the clues in the literature to hypothesize where the glutes might be on the curve.
First off, we’re quite confident that the glutes are at least active on the descending portion of the curve as we have studies showing that deep squats promote more glute growth than partial squats (Kubo et al., 2019). In addition, we know that the glutes exhibit a high level of activity in hip thrust or glute bridge exercises (Contreras et al. 2015) which would suggest that they’re likely not experiencing active insufficiency at short muscle lengths. In muscles that achieve active insufficiency, like the gastrocnemius, we see activation reduce at shorter muscle lengths (Hebert-Losier et al., 2012). This is why we see many physical therapy journals recommend hip thrust or glute bridge exercises to improve glute activation; not only do they activate the glutes well, but 2/3 of the hamstrings muscles will also be actively insufficient which further isolates the glutes (Buckthorpe et al., 2019).
Since the glutes are active at nearly all muscle lengths during training (Contreras et al., 2015; Contreras et al., 2016), I think it’s safe to hypothesize that the glutes are active on both the plateau and descending portion of the curve, but not the ascending portion. Therefore, use multiple exercises and multiple ranges of motion to train the glutes.
Calves
Last, but definitely not least, it’s time to discuss the calves. The calves are comprised of two muscles, the gastrocnemius and the soleus, which collectively form the, “triceps surae.” The calves are interesting as the soleus and gastroc actually occupy different portions of the length-tension curve, even though they perform the same actions. This is due to the fact that the gastrocnemius crosses the knee joint while the soleus does not.
As you can see in the soleus curve, the soleus is mostly active on the plateau and descending portions of the curve (Chen & Delp, 2016). However, the gastroc is shown in the colorful chart from Hoffman et al. (2013) in which we can see that it is only active on the plateau and ascending portions of the curve. Multiple other research groups have uncovered similar findings with the gastroc (Herzog et al., 1991; Maganaris, 2003) which lends strong support to the development of active insufficiency in this muscle.
So, what does this mean for training? The soleus can be mostly isolated using seated calf raises with a flexed knee. If you want to train the gastroc, you’re going to have to use a straight leg calf raise variation. In addition, the soleus should be trained at longer lengths (hold the stretch!) for best results. However, this stretching is likely to cause some soreness in the gastroc due to its position on the length-tension curve (Brockett et al., 2002).
Anatomical Cheat Sheet
At this point in writing this piece, it’s reasonable to assume that there’s been a bit of an information overload. How can we possibly expect you to remember each muscle group’s position on the curve? There’s actually a super easy cheat sheet that works for just about every muscle group: simply assume the “anatomical position” and move your joints. Can the muscle shorten or lengthen from that position? That usually dictates its spot on the curve!
For instance, the anatomical position for the biceps is with the elbow fully extended. With this in mind, the biceps only function on the ascending portion of the curve as they can only shorten. However, the shoulder is also in a neutral position, and from here we can both flex and horizontally adduct the shoulder and vice-versa. Therefore, the pecs can lengthen and shorten from this position, thus their place on all portions of the curve.
This “cheat sheet” also helps strengthen our assumptions about where the glutes might lie on the curve. From the anatomical position, you can absolutely lengthen the glutes through hip flexion. However, you can also hyperextend the hip to a degree, so it’s certainly possible that the glutes might travel down the ascending portion of the curve as well.
Any time you forget where a muscle is at, simply assume this position and see if a muscle shortens, lengthens, or both from this position. Annnnnd we just summed up the entire article in less than 250 words. Whoops.
Conclusion
As you many have noticed, we used true APA formatting for our in-text citations rather than the usual numbering system we use. While this makes the article look a little wordier (apologies), it’s necessary as we anticipate a decent number of updates to this article as we start to uncover more about the training application of the length-tension curve with each muscle group. Foregoing the number system makes it much easier to add edits and references.
With that out of the way, how do we conclude this piece? First of all, know that the length-tension role absolutely plays a role in muscle growth. However, much of what you may have learned in school only began to scrape the dust off of this concept. Not every muscle is on the same portion of the curve, so it’s necessary to understand the key concepts of the curve with each muscle group to optimize development and minimize wasted reps in the gym. Eventually, we’ll create some video lectures with this piece to help show more applicable uses of this knowledge in the gym.
For now, enjoy nerding out on the reading – we certainly enjoyed collecting references and writing this one.
References
- Bloomquist, K., Langberg, H., Karlsen, S., Madsgaard, S., Boesen, M., & Raastad, T. (2013). Effect of range of motion in heavy load squatting on muscle and tendon adaptations. European journal of applied physiology, 113(8), 2133-2142.
- Brockett, C. L., Morgan, D. L., Gregory, J. E., & Proske, U. (2002). Damage to different motor units from active lengthening of the medial gastrocnemius muscle of the cat. Journal of Applied Physiology, 92(3), 1104-1110.
- Buckthorpe, M., Stride, M., & Della Villa, F. (2019). Assessing and treating gluteus maximus weakness–a clinical commentary. International Journal of Sports Physical Therapy, 14(4), 655.
- Burkholder, T. J. (2007). Mechanotransduction in skeletal muscle. Frontiers in Bioscience: A Journal and Virtual Library, 12, 174.
- Chen, X., & Delp, S. L. (2016). Human soleus sarcomere lengths measured using in vivo microendoscopy at two ankle flexion angles. Journal of Biomechanics, 49(16), 4164-4167.
- Contreras, B., Vigotsky, A. D., Schoenfeld, B. J., Beardsley, C., & Cronin, J. (2015). A comparison of gluteus maximus, biceps femoris, and vastus lateralis electromyographic activity in the back squat and barbell hip thrust exercises. Journal of Applied Biomechanics, 31(6), 452-458.
- Contreras, B., Vigotsky, A. D., Schoenfeld, B. J., Beardsley, C., & Cronin, J. (2016). A comparison of gluteus maximus, biceps femoris, and vastus lateralis electromyography amplitude in the parallel, full, and front squat variations in resistance-trained females. Journal of Applied Biomechanics, 32(1), 16-22.
- Cutts, A. (1988). The range of sarcomere lengths in the muscles of the human lower limb. Journal of Anatomy, 160, 79.
- Garner, B. A., & Pandy, M. G. (2003). Estimation of musculotendon properties in the human upper limb. Annals of Biomedical Engineering, 31(2), 207-220.
- Gerling, M. E., & Brown, S. H. (2013). Architectural analysis and predicted functional capability of the human latissimus dorsi muscle. Journal of Anatomy, 223(2), 112-122.
- Goto, M., Maeda, C., Hirayama, T., Terada, S., Nirengi, S., Kurosawa, Y., … & Hamaoka, T. (2019). Partial range of motion exercise is effective for facilitating muscle hypertrophy and function through sustained intramuscular hypoxia in young trained men. The Journal of Strength & Conditioning Research, 33(5), 1286-1294.
- Hébert-Losier, K., Schneiders, A. G., García, J. A., Sullivan, S. J., & Simoneau, G. G. (2012). Influence of knee flexion angle and age on triceps surae muscle activity during heel raises. The Journal of Strength & Conditioning Research, 26(11), 3124-3133.
- Herzog, W., Read, L. J., & Ter Keurs, H. E. D. J. (1991). Experimental determination of force—length relations of intact human gastrocnemius muscles. Clinical Biomechanics, 6(4), 230-238.
- Hik, F., & Ackland, D. C. (2019). The moment arms of the muscles spanning the glenohumeral joint: a systematic review. Journal of Anatomy, 234(1), 1-15.
- Hoffman, B. W., Cresswell, A. G., Carroll, T. J., & Lichtwark, G. A. (2013). Muscle fascicle strains in human gastrocnemius during backward downhill walking. American Journal of Physiology-Heart and Circulatory Physiology.
- Kellis, E., Galanis, N., Kapetanos, G., & Natsis, K. (2012). Architectural differences between the hamstring muscles. Journal of Electromyography and Kinesiology, 22(4), 520-526.
- Koo, T. K., Mak, A. F., & Hung, L. K. (2002). In vivo determination of subject-specific musculotendon parameters: applications to the prime elbow flexors in normal and hemiparetic subjects. Clinical Biomechanics, 17(5), 390-399.
- Krüger, M., & Kötter, S. (2016). Titin, a central mediator for hypertrophic signaling, exercise-induced mechanosignaling and skeletal muscle remodeling. Frontiers in Physiology, 7, 76.
- Kubo, K., Ikebukuro, T., & Yata, H. (2019). Effects of squat training with different depths on lower limb muscle volumes. European Journal of Applied Physiology, 119(9), 1933-1942.
- Landin, D., Thompson, M., & Jackson, M. (2018). Functions of the triceps brachii in humans: a review. Journal of Clinical Medicine Research, 10(4), 290.
- Lieber, R. L., Roberts, T. J., Blemker, S. S., Lee, S. S., & Herzog, W. (2017). Skeletal muscle mechanics, energetics and plasticity. Journal of Neuroengineering and Rehabilitation, 14(1), 108.
- Maeo, S., Meng, H., Yuhang, W., Sakurai, H., Kusagawa, Y., Sugiyama, T., … & Isaka, T. (2020). Greater Hamstrings Muscle Hypertrophy but Similar Damage Protection after Training at Long versus Short Muscle Lengths. Medicine and Science in Sports and Exercise.
- Maganaris, C. N. (2003). Force‐length characteristics of the in vivo human gastrocnemius muscle. Clinical Anatomy: The Official Journal of the American Association of Clinical Anatomists and the British Association of Clinical Anatomists, 16(3), 215-223.
- Murdock, C. J., & Agyeman, K. (2020). Anatomy, Abdomen and Pelvis, Rectus Femoris Muscle. In StatPearls [Internet]. StatPearls Publishing.
- Murray, W. M., Buchanan, T. S., & Delp, S. L. (2000). The isometric functional capacity of muscles that cross the elbow. Journal of Biomechanics, 33(8), 943-952.
- Pinto, R. S., Gomes, N., Radaelli, R., Botton, C. E., Brown, L. E., & Bottaro, M. (2012). Effect of range of motion on muscle strength and thickness. The Journal of Strength & Conditioning Research, 26(8), 2140-2145.
- Sato, S., Yoshida, R., Kiyono, R., Yahata, K., Yasaka, K., Nunes, J. P., … & Nakamura, M. (2021). Elbow joint angles in elbow flexor unilateral resistance exercise training determine its effects on muscle strength and thickness of trained and non-trained arms. Frontiers in Physiology, 12.
- Schoenfeld, B. (2002). Accentuating muscular development through active insufficiency and passive tension. Strength & Conditioning Journal, 24(4), 20-22.
- Son, J., Indresano, A., Sheppard, K., Ward, S. R., & Lieber, R. L. (2018). Intraoperative and biomechanical studies of human vastus lateralis and vastus medialis sarcomere length operating range. Journal of Biomechanics, 67, 91-97.
- Stasinaki, A. N., Zaras, N., Methenitis, S., Tsitkanou, S., Krase, A., Kavvoura, A., & Terzis, G. (2018). Triceps brachii muscle strength and architectural adaptations with resistance training exercises at short or long fascicle length. Journal of Functional Morphology and Kinesiology, 3(2), 28.
- Wackerhage, H., Schoenfeld, B. J., Hamilton, D. L., Lehti, M., & Hulmi, J. J. (2019). Stimuli and sensors that initiate skeletal muscle hypertrophy following resistance exercise. Journal of Applied Physiology, 126(1), 30-43.
- Wan, X., Qu, F., Garrett, W. E., Liu, H., & Yu, B. (2017). Relationships among hamstring muscle optimal length and hamstring flexibility and strength. Journal of Sport and Health Science, 6(3), 275-282.
- Watanabe, K., Kouzaki, M., & Moritani, T. (2012). Task-dependent spatial distribution of neural activation pattern in human rectus femoris muscle. Journal of Electromyography and Kinesiology, 22(2), 251-258.
- Wolfe, S. W., Wickiewicz, T. L., & Cavanaugh, J. T. (1992). Ruptures of the pectoralis major muscle: an anatomic and clinical analysis. The American Journal of Sports Medicine, 20(5), 587-593.
- Wu, W., Lee, P. V., Bryant, A. L., Galea, M., & Ackland, D. C. (2016). Subject-specific musculoskeletal modeling in the evaluation of shoulder muscle and joint function. Journal of Biomechanics, 49(15), 3626-3634.
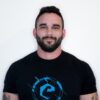
From being a mediocre athlete, to professional powerlifter and strength coach, and now to researcher and writer, Charlie combines education and experience in the effort to help Bridge the Gap Between Science and Application. Charlie performs double duty by being the Content Manager for The Muscle PhD as well as the Director of Human Performance at the Applied Science and Performance Institute in Tampa, FL. To appease the nerds, Charlie is a PhD candidate in Human Performance with a master’s degree in Kinesiology and a bachelor’s degree in Exercise Science. For more alphabet soup, Charlie is also a Certified Strength and Conditioning Specialist (CSCS), an ACSM-certified Exercise Physiologist (ACSM-EP), and a USA Weightlifting-certified performance coach (USAW).